A one-health approach to using sheep in research, with a focus on neuroscience studies
Abstract
Sheep have been an important animal for both academic and basic sciences education, with a positive impact on the public health sector and, subsequently, One Health. This review presents the impact of sheep on research with a specific focus on neuroscience studies. Disorders, as well as neuroendocrine and environmental factors affecting the brain, the spinal cord, and the peripheral nervous system, are selected, and relevant research and sheep models mimicking human diseases are described. The review discusses various sheep models, encompassing prion, Parkinson’s, Alzheimer’s, Huntington’s disease, and neuronal ceroid lipofuscinoses (Batten Disease), along with ischemic stroke. Sheep play a pivotal role in elucidating the pathogenesis and/or treatment for the aforementioned diseases. Furthermore, this research is underpinned by solid neuroanatomy knowledge. Consequently, we outline the main reasons why sheep are such robust research models. In conclusion, we demonstrate the important role that sheep models fulfill in advancing the mission of the One Health Initiative.
Keywords
INTRODUCTION
The One Health initiative centers around the tenet that humans, animals, and the environment are all integral parts of the same system impacting one another[1,2]. This tenet arose to address complicated interdisciplinary problems affecting these parts of the system[3]. Additionally, in the “One Health, One Medicine” concept, animals and humans develop diseases sharing a common etiology, pathology, and/or even related treatment, therefore establishing a link between them[4].
For millennia, sheep have been used as farm animals, providing both excellent nutrition and clothing to a growing human population, and strongly influencing the economy of countries[5]. Sheep research, with a One Health impact, has been conducted in various scientific fields, including infectious agents, like Ngari virus, that affects sheep and humans[6], antimicrobial resistance research[3], and comparative research between species, like the study of the mastitis microenvironment, leading to progress in human research[7]. Additionally, the cognitive capacity of the sheep makes them well-suited for the implementation of advanced technologies. For example, experiments using the ME20.4 immunoglobulin G (IgG)-saporin, a toxin that induces specific basal forebrain cholinergic (BFC) lesions, were conducted to investigate the role of the BFC system in social recognition training. Olfactory recognition of lambs by their mother serves as an appropriate model for employing this advanced “molecular surgery” technique[8,9].
Neuroscience research is undeniably dominated by rodents[10,11]. These small animals have numerous reasons for being the most commonly used model, which include: their low costs as laboratory animals, well-defined biology, fast reproductive rates, a plethora of available research tools, and above all, their genetic manipulation to better recapitulate the human condition[12,13]. Rodents provide detailed insight into the physiological aspects of human disease and are integral to human drug development. However, there is still a need for animal models with brain structures comparable to those of humans[10,11]. While non-human primates (NHPs) appear as the most relevant phylogenetic candidates for preclinical studies, research on them is fraught with ethical concerns[4,14,15]. Public opinion and disapproval of NHP research are based on the phenotypic/genetic proximity of NHPs to humans, their highly developed social skills, and many more characteristics[16].
It is noteworthy that sheep can fulfill a didactical role. In veterinary medicine, sheep are an established reference animal for anatomic and histologic education. Moreover, ovine models are being used in postgraduate fields outside the umbrella of veterinary medical education. Training human surgical techniques described by Ianacone et al. (2016) and Isaacson et al. (2022) are simulated in ovine tissue to model human surgical approaches[17,18]. Consequently, sheep provide an advantageous tool for translational research and educational purposes.
Many large animals have been used in neuroscience research: horses, cattle, pigs, sheep, dogs, cats, etc.[4,14,19]. Their use in research is a prerequisite since the U.S. Food and Drug Administration (FDA) requires toxicity tests carried out on both a rodent and a non-rodent species for novel human drug approval[20]. In this review, the authors emphasize sheep’s role in neuroscience research with reference to neurological disorders, spinal cord injuries, ischemic stroke, and neuroendocrine studies. Thus, sheep provide an emerging, convenient, and applicable disease model reflecting human disease due to neuroanatomical and physiological similarities.
NEUROLOGICAL DISORDERS
In line with the One Health notion, neuroscience studies on sheep are presented to deduce knowledge applicable mainly to humans, to either fill gaps regarding the nervous system’s function or collect neurologic disorder data and apply potential therapeutic strategies. The first disorder discussed here, though, ticks an extra One Health box[2], and helps to elucidate the prion agent’s transmission from sheep to humans[21] [Figure 1].
Figure 1. Sketch showing the utility of sheep as experimental models in a broad range of disciplines.All this research is grounded on a neuroanatomical basis.
Prion diseases
Prion disorders can affect many different mammals, from Scrapie in sheep to Creutzfeldt-Jakob disease in humans[22]. This fatal neurodegenerative disorder, known as Scrapie, is the result of the misfolding of the normal prion protein (PrPC) into the disease-associated form (PrPSc), and accumulation of this PrPSc form. The misfolding can be caused by a mutation in the PRNP gene, an infection with the misfolded protein, or a combination of both factors[21]. It entails a long asymptomatic subclinical phase/incubation period, followed by a comparatively shorter clinical phase leading to death[21-23]. Under certain conditions, Scrapie may pose a zoonotic threat by the consumption of prion-infected (PrPSc) material[21,23]. This explains the extra surveillance measures worldwide to detect the prevalence and geographical disease distribution in small ruminants and cattle in order to avoid further human exposure to prion agents[21].
Prions were first observed in sheep. Isolation of the scrapie strains from sheep has been characterized through experimental transmission studies to rodents[22,24]. Prion diseases are a well-known category of proteinopathies, which also includes other human neurological disorders such as Alzheimer’s disease (AD), Parkinson’s disease (PD), Huntington’s disease (HD), amyotrophic lateral sclerosis (ALS), Creutzfeldt-Jakob disease, and more. These proteinopathies may be influenced by different multifactorial aetiologies, but they commonly share the accumulation of abnormal proteins, which plays a key role in their pathogenesis[22,24-26]. Subsequently, a deeper understanding of Scrapie pathogenesis and treatment approaches may additionally prove beneficial for other proteinopathies[25].
The ability to cross the species barrier when BSE infection was orally transmitted to genetically susceptible sheep was demonstrated by Jeffrey et al. (2001). One hundred percent infection rate was observed, with abnormal prion accumulation detected 22 months post inoculation[27]. Previous studies showed that the initial accumulation of the infectious agent occurs in Peyer’s patch follicles. Therefore, Åkesson et al. (2011) conducted an experimental study in lambs, which involved surgically manipulating intestinal loops and then inoculating these isolated loops with scrapie brain pool inoculum, normal brain homogenate, or sucrose solution. They concluded that the intestinal epithelium remained undisturbed, and thus formed different hypotheses on the infection of these structures[28].
Few diagnostic tools exist for the detection of prion proteins. In 2015, an effort was made to create a Scrapie blood plasma test based on previous studies, indicating infectivity was present in the blood before CNS and viscera deposition, even three months post inoculation[29,30]. Ultimately, Lim et al. (2015) showed positive results on the detection of Scrapie-infected sheep using a multimer detection system[31].
It is important to highlight that non-prion nervous system infections (i.e., bacteria, viruses, etc.) may ignite or intensify human prion disorders[32]. This is particularly important in the post-COVID era, where a strong correlation between COVID-19 patients and prion-related diseases is emerging[33]. Therefore, understanding prion disease may pave the way for the development of novel therapeutic or prophylactic approaches. The use of sheep in this field of study is of paramount importance.
Parkinson’s disease
Parkinson’s disease causes a different kind of abnormal aggregated-protein deposition, where the misfolded a-synuclein causes the degeneration of dopaminergic neurons in the substantia nigra, leading mainly to motor function decline[24,26,34]. One study examines the ovine brain bioavailability of intranasally administered ropinirole. Rao et al. (2017) proved that their ropinirole nasal gel resulted in higher drug concentrations in sheep brains, suggesting a promising non-invasive alternative to intravenous injection[35]. Moreover, additional experiments using sheep as an intranasal drug bioavailability model continue to emerge.
Bourke (2018) proposes a molybdenum-deficient sheep PD model, causing symptoms of motoric and motor neuron dysfunction and dementia through astrocyte dysfunction[36]. The most commonly used PD model involves exposure to the neurotoxic agent, 1-methyl-4-phenyl-1,2,3,6-tetrahydropyridine (MPTP), which selectively targets PD-involved neurons[37,38]. This substance promotes the manifestation of PD symptoms in aged PD sheep model[39-41]. It is noteworthy that sheep, like humans, naturally develop age-related diseases, e.g., PD and AD[41].
Alzheimer’s disease
Furthermore, in aged sheep brains, neurofibrillary structures similar to those observed in human AD were observed[42]. Davies et al. (2022) have shown that humans and sheep have common AD pathological components, while Reid et al. (2017) demonstrated age-dependent plaque aggregation in sheep[43,44]. All the above strengthen sheep’s importance as an AD model.
Huntington’s disease
Mutant Huntingtin protein accumulation in brain neurons causes HD, a fatal genetic neurodegenerative disorder characterized by motor dysfunction and cognitive decline[45,46]. After many years of studying this disorder in rodents, the absence of an effective therapeutic agent against HD is leading to a shift in the use of large animal models. There are a number of scientific papers indicating the adoption of sheep as a more suitable HD model, based on sheep’s neuroanatomical similarities to the human brain, experimental convenience, and mainly for its longevity, allowing for long-term effect measurements of potential therapeutic agents[12,46-48]. Therefore, in 2010, a transgenic ovine (OVT73) HD model was developed[49]. This transgenic model, the OVT73 sheep, carries the human genetic variation, something that has not been achieved by the other transgenic HD models[47]. Studies on this sheep HD model demonstrate that, by five years of age, these animals exhibit brain pathology similar to that observed in human HD patients, and metabolic abnormalities, although without the neurodegenerative symptomatology, hinting at the diagnostic potential for presymptomatic human disease and indicating the potential for identifying novel biomarkers that can enhance the assessment of disease progression[45,47,50,51]. Another symptom of human HD is also exhibited in the sheep model: sleep disturbances. In general, HD causes presymptomatic circadian rhythm abnormalities, worsened with age in OVT73[52]. A sleep study using electroencephalography on HD and normal sheep revealed significant differences, indicating them as an important prognostic factor for HD patients[53]. A different study comparing normal and HD sheep showed elevated plasma melatonin levels in the latter, suggesting melatonin neuroprotective properties for huntingtin toxicity[54].
The development of this new transgenic ovine model revealed the lack of preclinical cognitive tests in sheep, which are necessary for assessing disease-related cognitive decline and, more importantly, the effect of potential treatments[55]. Morton et al. (2011) demonstrated sheep can perform executive cognitive tasks, making the cognitive decline of sheep in neurodegenerative diseases quantifiable[47,55]. Additionally, McBride et al. (2016) created a mobile semi-automated system to test the cognitive abilities of sheep and other animals[56].
Sheep MRI imaging revealed discrepancies between aged HD and normal sheep brains. These discrepancies allow for therapeutic potency testing in HD sheep models at the early disease stages, prior to the onset of evident clinical symptoms[46]. Moreover, the establishment of an OVT73 multi-omic dataset provides additional practical information that can be beneficial for future HD research[57]. Finally, researchers evaluated viral therapies in HD sheep. Mondo et al. (2018) have studied the neuronal uptake and distribution of three adeno-associated virus (AAV) therapies, while Pfister et al. (2018) showed that AAV9 can safely silence human mutant HD protein[58,59].
Neuronal ceroid lipofuscinosis (Batten disease)
The final neurological disorder in this review is Batten Disease (BD), also known as neuronal ceroid lipofuscinoses, which has been extensively studied on sheep. Batten Disease refers to a group of rare inherited neurodegenerative diseases with limited treatment options, stemming from dysfunctional storage disorders in lysosomes. Common symptomatology of this group of diseases includes dementia, visual impairment, seizures, and progressive motor decline, resulting in premature death[60-62]. In humans, there is also a rare juvenile manifestation of the disease linked with the CLN3 gene, occurring in children between the ages of 5 and 10, unfortunately, with no available treatment options. Patients present the common symptoms of the group and, in rare cases, heart malfunction, with the majority of individuals not exceeding a life expectancy beyond their early 20s[63,64]. Large animal models for this disease primarily include dogs and sheep[65,66]. Both genetically modified and naturally occurring sheep models are available[61,65-68].
The translational relevance of the CLN sheep, [referring to the family of mutated CLN (ceroid lipofuscinosis, neuronal) genes, which causes Batten disease] is amplified through several studies. Table 1 provides a concise summary of them:
Ovine animal models of BD
Mutation in gene | Breed | Occurrence | Experimental therapies | Onset of clinical symptoms | Examples of studies/model characteristics |
CLN1[68] | Texel-cross[69] | Gene editing | Enzyme Replacement Therapy[70] | N/A | It produces common phenotypical human disease characteristics |
CLN5[71] | New Zealand Borderdale | Natural | Gene Therapies[72,73] | 11-12 months[74] | In a comparative EEG study, CLN5 homozygous sheep present sleep abnormalities comparable to the brain activity of epileptic children[75] They manifest similarity to the molecular pathways of synapse pathophysiology with less developed animals (mouse and Drosophila models), allowing for target therapeutics on a larger animal[60] |
CLN6[71,76] | 1. New Zealand Borderdale 2. Australian Merino 3. New Zealand South Hampshire | Natural | 1. Gene Therapies 2. Gene Therapies 3. Gene Therapies, Anti-inflammatory drug[67] | 1. 6 months 2. 7-10 months 3. 10-14 months | A longitudinal MRI study in two different time points in South Hampshire sheep revealed atrophy in the cortex and other brain anatomical areas, which is a key point to observe the progression of the disease and alterations or deceleration caused by treatment[77] |
CLN10[78] | White Swedish Landrace | Natural | N/A | At birth[79] | Brain atrophy and early death are the result of a mutation in the cathepsin D gene, in this model of disease |
Therapeutic BD approaches include enzyme replacement therapy and gene therapy, both of which have been extensively studied in murine models, with limited translation potential in humans[66,80]. A cross-species approach by Nelvagal et al. (2022) treated mice and sheep with enzyme replacement therapy and obtained positive results when assessed with MRI and neuropathology[70]. Gene therapy was also applied to sheep through lentiviral or AAV injections[72]. These techniques slowed disease progression[73,81]. Finally, a neural cell culture from BD sheep showed a reversal of pathological storage body accumulation following the application of lentiviral gene therapy[82].
SPINAL CORD INJURY
Spinal cord injury (SCI) [Figure 1] refers to damage occurring directly by mechanical impact or indirectly by the body’s attempt to repair the damage over time. It can be inflicted by trauma, disease, degeneration, or ischemia[83-85]. This uncommon medical problem can lead to overwhelming financial costs arising from high rates of mortality, hospitalization, and patient care and the productivity loss of the affected individuals. Added costs arise from post-SCI complications, including neurological dysfunction like spasticity, paralysis, paraparesis and fatigue, and potential future contractures, pressure ulcers, and infections[83,86,87]. A deeper understanding of the biology, pathology, and post-SCI repair mechanisms is required due to the lack of a well-defined treatment. This knowledge deficit is driving researchers to a shift towards large animal models for better human translational outcomes[83].
Animal SCI models include rats, mice, rabbits, dogs, cats, pigs, and NHPs, while far fewer studies have been conducted in sheep. Although the most commonly used SCI animal model is the rat, its suitability for preliminary studies is uncertain[83,84,86]. The preference for rats in acute SCI studies has been questioned by Petteys et al. (2017). They hinted that the vast majority of rat SCI research has limited benefits for the human patient[86]. Advantages of using rodents include their cost-effectiveness, well-studied SCI biology, low ethical restrictions for research, and standardized methods to effectively replicate SCI conditions[83,84,86]. The disadvantages include significant anatomical differences between humans and rodents. In terms of size, rodent spinal cord is far shorter than that of humans. This size differential is vital for regenerative spinal cord studies because rodents require shorter nerve fiber regeneration distances; therefore, restoration of function is more probable in smaller species, impairing translational results[86,88]. Sheep have a large-diameter spinal cord, spine, and spinal cord anatomical similarities to humans; they have well-studied CNS electrophysiology, and follow similar injury and repair patterns as humans[85-87]. The ovine body size permits chronic human-scale device implantation for electrophysiological studies, playing a crucial role in implant testing[87]. Conclusively, large animal models, like sheep, more effectively approximate human SCIs.
There is a pivotal hurdle to overcome when studying sheep SCI. Many studies lack consistent approaches because, in contrast to rodents, sheep have not been widely studied and standardized sheep SCI methods do not exist[86]. In previous studies, a weighted drop object falling from a certain height was used onto the exposed spinal cord to cause its injury[89,90]. To overcome this problem, Petteys et al. (2017) created a controlled electromagnetic spinal cord impactor for large animals. This device will allow future therapeutical studies for acute traumatic SCI in sheep[86].
An important treatment for this injury is intradural or epidural spinal cord stimulation using electrodes to alleviate post-SCI chronic pain and spasticity. Sheep have been used to test the efficacy and safety of these implantable devices[91,92]. Several factors require consideration: proximity to the stimulation area, magnitude of the stimuli, durability and longevity of the device, and prevention of ischemic effects due to pressure from the device[91,92]. Safayi et al. (2015) have examined the gait of sheep walking on a treadmill, comparing sheep’s walking patterns before and after a SCI through video analysis, the method of which is applicable to future novel therapeutic assessment[90]. This has an observable One Health approach because it has already been applied to SCI intervention of other quadrupedal species[90].
Human spinal cord ischemia is a common sequelae to an accident or trauma requiring prompt action to restore oxygenation and blood supply to avoid secondary SCI[85]. An adult Dorset sheep model was used to develop a fiber optic device for monitoring spinal cord blood supply; such devices provide an early diagnostic tool for this damage[85]. In another study, Merino sheep were used to understand SCI pathophysiology following endovascular descending thoracic aortic surgery[93].
ISCHEMIC STROKE RESEARCH
The majority of human brain ischemia clinical cases are caused by vasculature blockage in the brain from ischemic Stroke (IS) [Figure 1][94]; As of May 2023, there is only one therapeutic agent for IS, called tissue plasminogen activator, according to the National Institute of Health. This sole medicinal contribution is extrapolated from rodent IS models[95,96]. To increase novel therapy research, large animal models are required. This is suggested by the Stroke Therapy Academic Industry before proceeding with clinical trials for Acute Ischemic Stroke[94,95]. Large animal IS models with high predictive value include NHPs, dogs, swine, sheep, and cats. Blood sampling is easier due to larger blood volumes, compared to rodents, which is very important for prognostic or recovery markers testing[95]. Additionally, there are plenty of anatomical and physiological similarities between sheep and humans, many of which are later reported in this review, like size, morphology, and gyrification.
Experimental IS models are either endovascular or surgical. A limiting factor for sheep endovascular IS studies is the rostral epidural rete mirabile (RM) formation, an anatomical structure that assists in selective brain cooling and body water balance maintenance, especially during high temperatures[97]. The RM is the result of many small-diameter arteries anastomoses between the maxillary and the internal carotid arteries, which prohibits the incidence of thromboembolic stroke and the required passage of microcatheters, allowing only for surgical approaches[94,95,97]. Boltze et al. (2008) described their results after permanent middle cerebral artery occlusion (MCAO) in sheep. They demonstrated that this novel focal cerebral ischemia model allows for controlled lesion size, with the analogous neural function effect, according to behavioral assessment. Positron emission tomography, MRI, and pathological, histological, and immunohistological testing revealed stroke-induced changes similar to human findings[94,98]. Wells et al. (2012) also verified the development of an ovine IS model while additionally comparing the effects between permanent and transient MCAO, using physiological and histopathological changes as well as infarct volumes in the brain. Both groups showed ischemic injury characteristics; the permanent occlusion group showed larger infarct volumes, whereas the second group, of aneurysm clip application, additionally allowed for future reperfusion studies[94,99]. Neither Boltze nor Wells reported mortality or complications during the experiments, and they both established sheep as a validated model in the stroke research field[98,99].
NEUROENDOCRINOLOGAL RESEARCH
Sheep play a crucial role in research associated with the discipline of neuroendocrinology [Figure 1], which orchestrates and regulates vital functions and physiological behaviors through the constant interactions between the nervous and endocrine systems. Neuroendocrinological research topics include the suppression of reproductive activity due to stress, undernutrition, and inflammation[100-103], and seasonal sheep neuroendocrine regulation[104,105]. Their outcomes assist in understanding mechanisms governing neuroendocrine human and ovine biology. More specifically, the regulation and control of ovine reproductive characteristics contribute to increased food and wool production[104,105]. In contrast to rodents, sheep show similarities in the mediation of progesterone negative feedback with primates during the luteal phase[106]. As previously mentioned, the large blood volumes, which allow for extensive serial sampling, provide repeated measurements of blood plasma to characterize the animal hormonal secretory profiles[104]. The direct hypophyseal portal blood sampling, coupled with jugular blood sampling, is another significant benefit. This can be achieved on unanesthetized and unrestrained/freely moving sheep for extended periods of time, omitting stress and anesthetic substances[104,107-109]. Even more importantly, the large size of the sheep brain is suitable for central agent administration, for example, intracerebroventricular norepinephrine injection, concomitant with frequent hypophyseal portal and peripheral blood sampling for extended periods[110]. Lastly, the ovine skull is suitable for chronic intubation through neurosurgical approaches, for repeated sampling of larger volumes of cerebrospinal fluid (CSF) compared to rodents, to counterbalance the physiologically low concentrations of substances found in CSF[111,112].
The works of Clarke, Goodman, Lehman, and other researchers utilize the above advantages to thoroughly study sheep hypothalamic, hypophyseal, and gonadal interactions[113-118]. These studies founded the hypothesis regarding the synchronized episodic gonadotropin-releasing hormone (GnRH) secretion[109]. The data acquired suggest that GnRH neuron activity is modulated by the KNDy neurons, containing kisspeptin, neurokinin B (NKB), and dynorphin. These neurons are located in the sheep arcuate nucleus of the medial basal hypothalamus, which is believed to play an essential role as the “GnRH pulse generator” similar to that in humans[119]. More recently, sheep research in this field has integrated the implementation of newer techniques in neuroanatomical analysis, such as RNAscope, a fluorescent in situ hybridization technique to assess mRNA at the cellular level[120]. For example, Merkley et al. (2020) and Harlow et al. (2022) have utilized this technique along with immunohistochemical analysis to examine KNDy cells in young male and female sheep during undernutrition, which align with a global impact of nutritional challenges in adolescents[121-123]. Another sheep study by Aerts et al. (2021) using RNAscope also utilized frequent blood sampling and immunohistochemistry to conclude that even though these neurons play an important role during pubertal development, they are not responsible for the initiation of puberty[124]. Moreover, kisspeptin, among other neuropeptides and neurotransmitters, is regulated by photoperiod, i.e., alterations in melatonin secretion, therefore linked to seasonal reproductive changes[125].
In the context of circadian rhythms and their importance in research, Esposito et al. (2020) experimented on rodents using three distinct cerebral ischemia therapeutic agents, yielding neuroprotective results. However, this study raised an issue regarding the translational potential due to the ineffectiveness of the same therapeutic scheme in humans[126]. Ultimately, they suggested that the opposite circadian rhythms between humans (diurnal) and rodents (nocturnal) need to be taken into consideration for translational studies on IS and CNS disease[126]. Research on sheep circadian rhythm is conclusive that they are mainly diurnal animals, like humans[127-129]. The latest technological approaches can be implemented in this field. For example, the use of GPS collars to extensively monitor sheep during free grazing can provide information on circadian rhythms in natural environments, which is nonapplicable in rodents[129]. Furthermore, Helm et al. (2013) proposed the potential seasonality of humans as an approach to better interpret human medical and psychological problems[130]. Therefore, it could be assumed that sheep circadian research might be more beneficial for human health.
Furthermore, oxytocin secretion is one of the major neuroendocrine mechanisms in mammals. Sheep as an animal model contributes vastly to the relevant research and the neuroanatomical basis of the milk ejection reflex has been elucidated[131,132]. Electrophysiological studies also exist to describe the milk-ejection reflex in sheep[133], where oxytocin is released only once or twice during each suckling session. Based on these data, modeling of oxytocin release in sheep has also been published[134], and the Artificial Neural Network produced by this work could be used for training on physiological data, leading to the improvement of milk production.
From a different perspective, the gut microbiome is shown to affect many physiological functions, including the neuroendocrine system of different animal species, establishing a microbiome-gut-brain axis (MGBA). In addition, MGBA affects the hypothalamic-pituitary-adrenal axis, as elucidated in microbiota-deficient germ-free animals[135,136]. The ovine gut microbiome composition has been studied through DNA/RNA sequencing techniques and compared to other polygastric and monogastric animals[137]. Gut microbiome metabolome studies were performed on Dorper and Tan sheep. Results on the performance of these animals were affected by microbiome composition and distinctive differences between the fermentation patterns of these two breeds were shown[138]. Conclusively, gut microbiome studies on sheep are extensive but restricted to animal production performance, mostly overrunning the MGBA[135].
ENVIRONMENTAL FACTORS WITH IMPACT ON THE NERVOUS SYSTEM
Finally, it is known that there are environmental factors [Figure 1]; (both to sheep and humans) such as bisphenols, affecting the function of many animal body systems, even from the embryonic stage[138]. For this reason, nutritional experiments were performed from earlier years in order to study the effects of macroelements, e.g., ammonium metavanadate, on lambs’ diet (mineral concentrations in animal tissues)[139]. In another study, sheep were given organophosphorus compounds and their subsequent neurological effects were monitored[140].
In later years, a sheep study has shown the results from nutritional exposure in biosolids containing heavy metals, which were associated with altered expression of GnRH, GnRH receptors, galanin receptors, and kisspeptin mRNA within the hypothalamus and pituitary gland. The effects of biosolid exposure at the hypothalamic level have concentrated on the GnRH neurosecretory system, as this is the primary system through which the reproductive axis is controlled and it is sensitive to the effects of endogenous and exogenous steroids both during development and in adult life. In most cases, these anatomical and functional differences do not result in altered peripheral hormone concentrations or reproductive function (like the lambing rate), indicating physiological compensation under the conditions tested[141].
In another study, gestational sheep exposure to a low dose of bisphenol A (BPA) is shown to affect maternofetal thyroid function and fetal brain development in a region-specific manner. The authors state that the use of a larger animal model enabled the examination of specific brain areas, leading to the generation of evidence indicating distinct region-specific effects of fetal BPA exposure on the brain metabolome[142].
Lastly, a publication focused on the results of heavy metal consumption (Cd, Pb, Cu, and Zn) both on humans and animals due to their vicinity in a smelting facility, implementing the One Health Initiative. Although it does not present effects on animals’ and humans’ nervous systems, it evaluates the contamination of pastures and farmlands with heavy metals and, in this regard, identifies the necessity of equivalent research to benefit both humans and animals[143].
FINAL REMARKS
In brief, we summarize the points that make sheep an essential animal model.
Why are sheep an important animal research model?[4,41,144-146]
1. Their docile nature permits easier and stress-free handling, saving time and money (e.g., purchase of restraints).
2. They have a size similar to humans, making sheep good candidates for:
(1) use of imaging techniques (e.g., MRI, CT)
(2) testing of medical devices (e.g., stents, pacemakers, heart valves)
(3) testing for surgical trials
(4) human-scale implantation electrophysiological tests
3. They are anatomically, physiologically, and genetically closer to humans than rodents.
4. They are relatively cost-efficient and easy to maintain compared to other large animals.
5. Their use as animal models is ethically more acceptable compared to NHPs and dogs.
6. Their long lifespan:
(1) allows for age-related studies (Scrapie)
(2) is more similar to macaque monkeys (10-30 years)
(3) requires longer studies for data accumulation compared to rodents
(4) may result in additional risk of population mortality in studies
7. They have naturally occurring diseases, similar to human diseases (BD).
8. They play an important role in preclinical trials.
9. They have a fully sequenced genome providing additional information.
10. Genome engineering is feasible in sheep, even supporting CRISPR/Cas9 to generate disease models.
11. They are relatively outbred, providing diverse populations for research, showing a wider spectrum of responses, and reflecting human heterogeneity.
12. There are available behavioral and cognitive tests.
13. They have a low litter size like humans.
Although there are reviews on the importance of sheep as an experimental animal, such as the one by Murray and Mitchell (2022)[147], our manuscript introduces sheep into the One Health system. We also believe the neuroanatomical similarities between humans and sheep are decisive for translational research. The similarities below are particularly relevant in establishing animal models.
Neuroanatomical and physiological significance of the sheep
The sheep brain anatomy, specifically its structure and organization, has significant similarities to NHPs and humans including[55,146,148-150]:
1. large brains
2. thick and well-developed meninges
3. human-like basal ganglia
(1) a dorsal striatum divided to caudate nucleus and putamen
(2) anatomical and neurochemical similarities of the substantia nigra
4. gyrencephalic cerebral cortexes
5. a brain with the capacity to remember the faces of other sheep and even humans.
6. white matter distribution like humans
7. well-defined sulci and gyri
8. relatively round skull, like humans, despite their elongated brain
9. lobulated cortexes with human-like landmarks
10. a brain region responsible for decision-making and emotional control similar to the corresponding human orbitofrontal cortex
What are some limitations when using sheep in neuroscience research?[4,20,147]
An extensive list of all the limitations and disadvantages of sheep in neuroscience research would result in a lengthy and time-consuming read. Nonetheless, the reader who is inexperienced with their use should get a general idea of the hurdles of using this large animal model. This includes:
1. higher expenses resulting from:
(1) housing facilities that are specialized for their larger body size, including proper surgical/operating facilities, imaging rooms, and specialized husbandry. Regarding the infrastructure needed for the housing of sheep in scientific institutions, more detailed and thorough guidelines can be found, for example, by the Animal Ethics Infolink online[151].
(2) a high skilled team of specialists for operations and surgeries, e.g., veterinarian assistance
(3) increasing maintenance expenses for long-term studies
(4) biosecurity measures and practices (examples listed below)[152,153]:
· quarantine/ acclimatization zones for animals before entering the main facility, including separate utensils and tools used only in this area
· vaccination, prophylactic treatment (anthelmintics), infectious disease testing
· isolation area for animals that are sick or suspected of having a disease, including separate utensils and tools used only in this area
· manure should be composted for at least a year before spreading on the environment
· the average costs of obtaining and keeping sheep as laboratory animals varies greatly between countries and different regions. In the references listed here, including the supplemental material by Taha et al. (2022), some indicative costs may provide the reader with a general idea of the expenses[95,154].
2. difficulty in finding and selecting proper antibodies and/or reagents for routine techniques, which are often more costly than rodents.
3. more time required for the accumulation of data, due to seasonality and longer gestation periods and lifespans.
4. anatomical differences to humans:
(1) due to their quadrupedal walk, they have a different neuraxis than humans, which may affect the outcomes of SCI models.
(2) regarding their gastrointestinal anatomy, which may play an important role in research about the MGBA.
(3) the presence of the rostral epidural rete mirabile, hindering endovascular IS research.
5. less available neurological and cognitive tests for sheep, compared to rodents.
CONCLUSION
This review serves to challenge the world’s general idea that sheep are low-intelligence farm animals, providing a significant contribution to neuroscience. The increased utilization of experimental techniques in several research disciplines (e.g., EEG) demonstrates that sheep are more cognitively robust than previously thought. Moreover, several naturally occurring diseases and novel gene-manipulating technologies for disease induction, in combination with innate neuroanatomical and physiological properties of the sheep that approximate those of humans, underscore the importance of sheep, not only for neuroscience research contributions but biomedical research in general. The conclusions drawn from these sheep experiments will allow for a significant understanding of disease mechanisms, and hopefully result in the advancement of therapeutic tools for human patients. Definitively, sheep research translational outcomes strengthen the One Health notion.
DECLARATIONS
AcknowledgmentWe thank Dr. Patrick Sharp, Attending Staff Veterinarian, University of California, Merced, USA for the critical review of the main part of the manuscript, and Giannis Graidis for capturing and editing the sheep photos used in the graphical abstract and Figure 1. The sheep photos are from the Farm Animal Clinic - Kolchiko, School of Veterinary Medicine, Aristotle University of Thessaloniki.
Authors’ contributionsDesign, writing, and editing of the manuscript: Chatzimanou T, Tsingotjidou A
Availability of data and materialsNot applicable.
Financial support and sponsorshipNone.
Conflicts of interestAll authors declared that there are no conflicts of interest.
Ethical approval and consent to participateNot applicable.
Consent for publicationNot applicable.
Copyright© The Author(s) 2023.
REFERENCES
2. Sinclair JR. Importance of a One Health approach in advancing global health security and the sustainable development goals. Rev Sci Tech Off Int Epiz 2019;38:145-54.
3. Gray GC, Mazet JAK. To Succeed, One Health must win animal agriculture’s stronger collaboration. Clin Infect Dis 2020;70:535-7.
4. Ribitsch I, Baptista PM, Lange-Consiglio A, et al. Large animal models in regenerative medicine and tissue engineering: to do or not to do. Front Bioeng Biotechnol 2020;8:972.
5. Whatford L, van Winden S, Häsler B. A systematic literature review on the economic impact of endemic disease in UK sheep and cattle using a One Health conceptualisation. Prev Vet Med 2022;209:105756.
6. Omoga DCA, Tchouassi DP, Venter M, et al. Circulation of ngari virus in livestock, kenya. mSphere 2022;7:e0041622.
7. Hughes K, Watson CJ. The mammary microenvironment in mastitis in humans, dairy ruminants, rabbits and rodents: a one health focus. J Mammary Gland Biol Neoplasia 2018;23:27-41.
8. Ancheta LR, Shramm PA, Bouajram R, Higgins D, Lappi DA. Saporin as a commercial reagent: its uses and unexpected impacts in the biological sciences-tools from the plant kingdom. Toxins 2022;14:184.
9. Ferreira G, Meurisse M, Tillet Y, Lévy F. Distribution and co-localization of choline acetyltransferase and p75 neurotrophin receptors in the sheep basal forebrain: implications for the use of a specific cholinergic immunotoxin. Neuroscience 2001;104:419-39.
10. Ellenbroek B, Youn J. Rodent models in neuroscience research: is it a rat race? Dis Model Mech 2016;9:1079-87.
11. Keifer J, Summers CH. Putting the “Biology” back into “Neurobiology”: the strength of diversity in animal model systems for neuroscience research. Front Syst Neurosci 2016;10:69.
12. Morton AJ, Howland DS. Large genetic animal models of Huntington’s disease. J Huntingtons Dis 2013;2:3-19.
13. Ziegler A, Gonzalez L, Blikslager A. Large animal models: the key to translational discovery in digestive disease research. Cell Mol Gastroenterol Hepatol 2016;2:716-24.
14. Eaton SL, Wishart TM. Bridging the gap: large animal models in neurodegenerative research. Mamm Genome 2017;28:324-37.
15. Grow DA, McCarrey JR, Navara CS. Advantages of nonhuman primates as preclinical models for evaluating stem cell-based therapies for Parkinson’s disease. Stem Cell Res 2016;17:352-66.
16. European Commission. Directorate General for Health and Food Safety. Final opinion on the need for non-human primates in biomedical research, production and testing of products and devices (Update 2017). Available from: https://data.europa.eu/doi/10.2875/337906. [Last accessed on 25 Oct 2023].
17. Isaacson G, Wulc AE. Applicability of a sheep model for training in plastic surgery of eyelids and orbit. Ear Nose Throat J 2022;101:43S-9S.
18. Ianacone DC, Gnadt BJ, Isaacson G. Ex vivo ovine model for head and neck surgical simulation. Am J Otolaryngol 2016;37:272-8.
19. Dolezalova D, Hruska-Plochan M, Bjarkam CR, et al. Pig models of neurodegenerative disorders: utilization in cell replacement-based preclinical safety and efficacy studies. J Comp Neurol 2014;522:2784-801.
20. Banstola A, Reynolds JNJ. The sheep as a large animal model for the investigation and treatment of human disorders. Biology 2022;11:1251.
21. Houston F, Andréoletti O. Animal prion diseases: the risks to human health. Brain Pathol 2019;29:248-62.
22. Block AJ, Bartz JC. Prion strains: shining new light on old concepts. Cell Tissue Res 2023;392:113-33.
23. Onodera T, Sakudo A. Introduction to current progress in advanced research on prions. Curr Issues Mol Biol 2020;36:63-6.
24. Ayers JI, Paras NA, Prusiner SB. Expanding spectrum of prion diseases. Emerg Top Life Sci 2020;4:155-67.
25. Carlson GA, Prusiner SB. How an infection of sheep revealed prion mechanisms in Alzheimer’s disease and other neurodegenerative disorders. Int J Mol Sci 2021;22:4861.
26. Wagner J, Ryazanov S, Leonov A, et al. Anle138b: a novel oligomer modulator for disease-modifying therapy of neurodegenerative diseases such as prion and Parkinson’s disease. Acta Neuropathol 2013;125:795-813.
27. Jeffrey M, Ryder S, Martin S, et al. Oral inoculation of sheep with the agent of bovine spongiform encephalopathy (BSE). 1. Onset and distribution of disease-specific PrP accumulation in brain and viscera. J Comp Pathol 2001;124:280-9.
28. Åkesson CP, McGovern G, Dagleish MP, et al. Exosome-producing follicle associated epithelium is not involved in uptake of PrPd from the gut of sheep (Ovis aries): an ultrastructural study. PLoS One 2011;6:e22180.
29. Sisó S, Jeffrey M, Houston F, Hunter N, Martin S, González L. Pathological phenotype of sheep scrapie after blood transfusion. J Comp Pathol 2010;142:27-35.
30. Lacroux C, Vilette D, Fernández-Borges N, et al. Prionemia and leukocyte-platelet-associated infectivity in sheep transmissible spongiform encephalopathy models. J Virol 2012;86:2056-66.
31. Lim K, Kim SY, Lee B, et al. Magnetic microparticle-based multimer detection system for the detection of prion oligomers in sheep. Int J Nanomedicine 2015;10:241-50.
32. Danics K, Forrest SL, Kapas I, et al. Neurodegenerative proteinopathies associated with neuroinfections. J Neural Transm 2021;128:1551-66.
33. Lukiw WJ, Jaber VR, Pogue AI, Zhao Y. SARS-CoV-2 invasion and pathological links to prion disease. Biomolecules 2022;12:1253.
35. Rao M, Agrawal DK, Shirsath C. Thermoreversible mucoadhesive in situ nasal gel for treatment of Parkinson’s disease. Drug Dev Ind Pharm 2017;43:142-50.
36. Bourke CA. Astrocyte dysfunction following molybdenum-associated purine loading could initiate Parkinson’s disease with dementia. NPJ Parkinsons Dis 2018;4:7.
38. Fornai F, Schlüter OM, Lenzi P, et al. Parkinson-like syndrome induced by continuous MPTP infusion: convergent roles of the ubiquitin-proteasome system and alpha-synuclein. Proc Natl Acad Sci U S A 2005;102:3413-8.
39. Hammock BD, Beale AM, Work T, et al. A sheep model for MPTP induced Parkinson-like symptoms. Life Sci 1989;45:1601-8.
40. Baskin DS, Browning JL, Widmayer MA, Zhu ZQ, Grossman RG. Development of a model for Parkinson’s disease in sheep using unilateral intracarotid injection of MPTP via slow continuous infusion. Life Sci 1994;54:471-9.
41. Capucciati A, Zucca FA, Monzani E, Zecca L, Casella L, Hofer T. Interaction of neuromelanin with xenobiotics and consequences for neurodegeneration; promising experimental models. Antioxidants 2021;10:824.
42. Nelson PT, Greenberg SG, Saper CB. Neurofibrillary tangles in the cerebral cortex of sheep. Neurosci Lett 1994;170:187-90.
43. Reid SJ, Mckean NE, Henty K, et al. Alzheimer’s disease markers in the aged sheep (Ovis aries). Neurobiol Aging 2017;58:112-9.
44. Davies ES, Morphew RM, Cutress D, Morton AJ, McBride S. Characterization of microtubule-associated protein tau isoforms and Alzheimer’s disease-like pathology in normal sheep (ovis aries): relevance to their potential as a model of Alzheimer’s disease. Cell Mol Life Sci 2022;79:560.
45. Skene DJ, Middleton B, Fraser CK, et al. Metabolic profiling of presymptomatic Huntington’s disease sheep reveals novel biomarkers. Sci Rep 2017;7:43030.
46. Taghian T, Gallagher J, Batcho E, et al. Brain alterations in aged OVT73 sheep model of Huntington’s disease: an MRI based approach. J Huntingtons Dis 2022;11:391-406.
47. Morton AJ. Large-brained animal models of Huntington’s disease: sheep. In: Precious SV, Rosser AE, Dunnett SB, editors. Huntington’s Disease. New York: Springer; 2018. p. 221-39.
48. Howland DS, Munoz-Sanjuan I. Mind the gap: models in multiple species needed for therapeutic development in Huntington’s disease. Mov Disord 2014;29:1397-403.
49. Jacobsen JC, Bawden CS, Rudiger SR, et al. An ovine transgenic Huntington’s disease model. Hum Mol Genet 2010;19:1873-82.
50. Handley RR, Reid SJ, Patassini S, et al. Metabolic disruption identified in the Huntington’s disease transgenic sheep model. Sci Rep 2016;6:20681.
51. Reid SJ, Patassini S, Handley RR, et al. Further molecular characterisation of the OVT73 transgenic sheep model of Huntington’s disease identifies cortical aggregates. J Huntingtons Dis 2013;2:279-95.
52. Morton AJ, Rudiger SR, Wood NI, et al. Early and progressive circadian abnormalities in Huntington’s disease sheep are unmasked by social environment. Hum Mol Genet 2014;23:3375-83.
53. Vas S, Nicol AU, Kalmar L, Miles J, Morton AJ. Abnormal patterns of sleep and EEG power distribution during non-rapid eye movement sleep in the sheep model of Huntington’s disease. Neurobiol Dis 2021;155:105367.
54. Morton AJ, Middleton B, Rudiger S, Bawden CS, Kuchel TR, Skene DJ. Increased plasma melatonin in presymptomatic Huntington disease sheep (Ovis aries): Compensatory neuroprotection in a neurodegenerative disease? J Pineal Res 2020;68:e12624.
56. McBride SD, Perentos N, Morton AJ. A mobile, high-throughput semi-automated system for testing cognition in large non-primate animal models of Huntington disease. J Neurosci Methods 2016;265:25-33.
57. Mears ER, Handley RR, Grant MJ, et al. A multi-omic Huntington’s disease transgenic sheep-model database for investigating disease pathogenesis. J Huntingtons Dis 2021;10:423-34.
58. Mondo E, Moser R, Gao G, et al. Selective neuronal uptake and distribution of AAVrh8, AAV9, and AAVrh10 in sheep after intra-striatal administration. J Huntingtons Dis 2018;7:309-19.
59. Pfister EL, DiNardo N, Mondo E, et al. Artificial miRNAs reduce human mutant huntingtin throughout the striatum in a transgenic sheep model of Huntington’s disease. Hum Gene Ther 2018;29:663-73.
60. Amorim IS, Mitchell NL, Palmer DN, et al. Molecular neuropathology of the synapse in sheep with CLN5 Batten disease. Brain Behav 2015;5:e00401.
61. Frugier T, Mitchell NL, Tammen I, et al. A new large animal model of CLN5 neuronal ceroid lipofuscinosis in Borderdale sheep is caused by a nucleotide substitution at a consensus splice site (c.571+1G>A) leading to excision of exon 3. Neurobiol Dis 2008;29:306-15.
62. Nelvagal HR, Lange J, Takahashi K, Tarczyluk-Wells MA, Cooper JD. Pathomechanisms in the neuronal ceroid lipofuscinoses. Biochim Biophys Acta Mol Basis Dis 2020;1866:165570.
63. Handrup MM, Mølgaard H, Andersen BN, Ostergaard JR. Pacemaker implantation in juvenile neuronal ceroid lipofuscinosis (CLN3)-a long-term follow-up study. Front Neurol 2022;13:846240.
64. Batten Disease News. Juvenile Batten Disease. Available from: https://battendiseasenews.com/juvenile-batten-disease/. [Last accessed on 25 Oct 2023].
65. Huber RJ, Hughes SM, Liu W, Morgan A, Tuxworth RI, Russell C. The contribution of multicellular model organisms to neuronal ceroid lipofuscinosis research. Biochim Biophys Acta Mol Basis Dis 2020;1866:165614.
66. Nelvagal HR, Cooper JD. Translating preclinical models of neuronal ceroid lipofuscinosis: progress and prospects. Expert Opinion on Orphan Drugs 2017;5:727-40.
67. Palmer DN, Neverman NJ, Chen JZ, et al. Recent studies of ovine neuronal ceroid lipofuscinoses from BARN, the Batten Animal Research Network. Biochim Biophys Acta 2015;1852:2279-86.
68. Eaton SL, Proudfoot C, Lillico SG, et al. CRISPR/Cas9 mediated generation of an ovine model for infantile neuronal ceroid lipofuscinosis (CLN1 disease). Sci Rep 2019;9:9891.
69. Mitchell NL, Russell KN, Barrell GK, Tammen I, Palmer DN. Characterization of neuropathology in ovine CLN5 and CLN6 neuronal ceroid lipofuscinoses (Batten disease). Dev Neurobiol 2023;83:127-42.
70. Nelvagal HR, Eaton SL, Wang SH, et al. Cross-species efficacy of enzyme replacement therapy for CLN1 disease in mice and sheep. J Clin Invest 2022;132:e163107.
71. Murray SJ, Almuqbel MM, Felton SA, et al. Progressive MRI brain volume changes in ovine models of CLN5 and CLN6 neuronal ceroid lipofuscinosis. Brain Commun 2023;5:fcac339.
72. kleine Holthaus S, Smith AJ, Mole SE, Ali RR. Gene therapy approaches to treat the neurodegeneration and visual failure in neuronal ceroid lipofuscinoses. In: Ash JD, Anderson RE, Lavail MM, Bowes Rickman C, Hollyfield JG, Grimm C, editors. Retinal Degenerative Diseases. Cham: Springer International Publishing; 2018. p. 91-9.
74. Mitchell NL, Murray SJ, Wellby MP, et al. Long-term safety and dose escalation of intracerebroventricular CLN5 gene therapy in sheep supports clinical translation for CLN5 Batten disease. Front Genet 2023;14:1212228.
75. Perentos N, Martins AQ, Watson TC, et al. Translational neurophysiology in sheep: measuring sleep and neurological dysfunction in CLN5 Batten disease affected sheep. Brain 2015;138:862-74.
76. Barry LA, Kay GW, Mitchell NL, Murray SJ, Jay NP, Palmer DN. Aggregation chimeras provide evidence of in vivo intercellular correction in ovine CLN6 neuronal ceroid lipofuscinosis (Batten disease). PLoS One 2022;17:e0261544.
77. Sawiak SJ, Perumal SR, Rudiger SR, et al. Rapid and progressive regional brain atrophy in CLN6 Batten disease affected sheep measured with longitudinal magnetic resonance imaging. PLoS One 2015;10:e0132331.
78. Tyynelä J, Sohar I, Sleat DE, et al. A mutation in the ovine cathepsin D gene causes a congenital lysosomal storage disease with profound neurodegeneration. EMBO J 2000;19:2786-92.
79. Cronin GM, Beganovic DF, Sutton AL, Palmer D, Thomson PC, Tammen I. Manifestation of neuronal ceroid lipofuscinosis in Australian Merino sheep: observations on altered behaviour and growth. Appl Anim Behav Sci 2016;175:32-40.
80. Johnson TB, Cain JT, White KA, Ramirez-Montealegre D, Pearce DA, Weimer JM. Therapeutic landscape for Batten disease: current treatments and future prospects. Nat Rev Neurol 2019;15:161-78.
81. Mitchell NL, Russell KN, Wellby MP, et al. Longitudinal in vivo monitoring of the CNS demonstrates the efficacy of gene therapy in a sheep model of CLN5 Batten disease. Mol Ther 2018;26:2366-78.
82. Hughes SM, Hope KM, Xu JB, Mitchell NL, Palmer DN. Inhibition of storage pathology in prenatal CLN5-deficient sheep neural cultures by lentiviral gene therapy. Neurobiol Dis 2014;62:543-50.
83. Sharif-Alhoseini M, Khormali M, Rezaei M, et al. Animal models of spinal cord injury: a systematic review. Spinal Cord 2017;55:714-21.
84. Wilson S, Nagel SJ, Frizon LA, et al. The hemisection approach in large animal models of spinal cord injury: overview of methods and applications. J Invest Surg 2020;33:240-51.
85. Mesquita RC, D’Souza A, Bilfinger TV, et al. Optical monitoring and detection of spinal cord ischemia. PLoS One 2013;8:e83370.
86. Petteys RJ, Spitz SM, Syed H, et al. Design and testing of a controlled electromagnetic spinal cord impactor for use in large animal models of acute traumatic spinal cord injury. J Clin Neurosci 2017;43:229-34.
87. Wilson S, Abode-Iyamah KO, Miller JW, et al. An ovine model of spinal cord injury. J Spinal Cord Med 2017;40:346-60.
88. Kwon BK, Oxland TR, Tetzlaff W. Animal models used in spinal cord regeneration research. Spine 2002;27:1504-10.
89. Yeo JD, Payne W, Hinwood B, Kidman AD. The experimental contusion injury of the spinal cord in sheep. Paraplegia 1975;12:279-98.
90. Safayi S, Jeffery ND, Shivapour SK, et al. Kinematic analysis of the gait of adult sheep during treadmill locomotion: parameter values, allowable total error, and potential for use in evaluating spinal cord injury. J Neurol Sci 2015;358:107-12.
91. Dalm BD, Viljoen SV, Dahdaleh NS, et al. Revisiting intradural spinal cord stimulation: an introduction to a novel intradural spinal cord stimulation device. Innovative Neurosurgery 2014;2:13-20. Available from: https://www.researchgate.net/profile/Sina-Safayi/publication/269392974_Revisiting_intradural_spinal_cord_stimulation_An_introduction_to_a_novel_intradural_spinal_cord_stimulation_device/links/548863560cf2ef3447908d46/Revisiting-intradural-spinal-cord-stimulation-An-introduction-to-a-novel-intradural-spinal-cord-stimulation-device.pdf. [Last accessed on 25 Oct 2023]
92. Flouty OE, Oya H, Kawasaki H, et al. Intracranial somatosensory responses with direct spinal cord stimulation in anesthetized sheep. PLoS One 2013;8:e56266.
93. Böckler D, Kotelis D, Kohlhof P, et al. Spinal cord ischemia after endovascular repair of the descending thoracic aorta in a sheep model. Eur J Vasc Endovasc Surg 2007;34:461-9.
94. Kaiser EE, West FD. Large animal ischemic stroke models: replicating human stroke pathophysiology. Neural Regen Res 2020;15:1377-87.
95. Taha A, Bobi J, Dammers R, et al. Comparison of large animal models for acute ischemic stroke: which model to use? Stroke 2022;53:1411-22.
96. NHLBI, NIH. Stroke. Treatment. Available from: https://www.nhlbi.nih.gov/health/stroke/treatment. [Last accessed on 25 Oct 2023].
97. Graczyk S, Zdun M. The structure of the rostral epidural rete mirabile in selected representatives of the Cervidae and Bovidae families. Acta Zoologica 2021;102:496-501.
98. Boltze J, Förschler A, Nitzsche B, et al. Permanent middle cerebral artery occlusion in sheep: a novel large animal model of focal cerebral ischemia. J Cereb Blood Flow Metab 2008;28:1951-64.
99. Wells AJ, Vink R, Blumbergs PC, et al. A surgical model of permanent and transient middle cerebral artery stroke in the sheep. PLoS One 2012;7:e42157.
100. Breen KM, Karsch FJ. New insights regarding glucocorticoids, stress and gonadotropin suppression. Front Neuroendocrinol 2006;27:233-45.
101. Karsch FJ, Battaglia DF, Breen KM, Debus N, Harris TG. Mechanisms for ovarian cycle disruption by immune/inflammatory stress. Stress 2002;5:101-12.
102. Tilbrook AJ, Turner AI, Clarke IJ. Effects of stress on reproduction in non-rodent mammals: the role of glucocorticoids and sex differences. Rev Reprod 2000;5:105-13.
103. Merkley CM, Shuping SL, Nestor CC. Neuronal networks that regulate gonadotropin-releasing hormone/luteinizing hormone secretion during undernutrition: evidence from sheep. Domest Anim Endocrinol 2020;73:106469.
104. Karsch FJ, Moenter SM. Neuroendocrine regulation of seasonal breeding cycles in the ewe. J Exp Zool Suppl 1990;256:17-21.
105. Clarke IJ. Sex and season are major determinants of voluntary food intake in sheep. Reprod Fertil Dev 2001;13:577-82.
106. Weems PW, Lehman MN, Coolen LM, Goodman RL. Chapter Four - The roles of neurokinins and endogenous opioid peptides in control of pulsatile LH secretion. Vitam Horm 2018;107:89-135.
107. Veldhuis JD, Fletcher TP, Gatford KL, Egan AR, Clarke IJ. Hypophyseal-portal somatostatin (SRIH) and jugular venous growth hormone secretion in the conscious unrestrained ewe. Neuroendocrinology 2002;75:83-91.
108. Frohman LA, Downs TR, Clarke IJ, Thomas GB. Measurement of growth hormone-releasing hormone and somatostatin in hypothalamic-portal plasma of unanesthetized sheep. Spontaneous secretion and response to insulin-induced hypoglycemia. J Clin Invest 1990;86:17-24.
109. Lehman MN, Coolen LM, Goodman RL. Importance of neuroanatomical data from domestic animals to the development and testing of the KNDy hypothesis for GnRH pulse generation. Domest Anim Endocrinol 2020;73:106441.
110. Engler D, Pham T, Fullerton MJ, Ooi G, Funder JW, Clarke IJ. Studies of the secretion of corticotropin-releasing factor and arginine vasopressin into the hypophysial-portal circulation of the conscious sheep. I. Effect of an audiovisual stimulus and insulin-induced hypoglycemia. Neuroendocrinology 1989;49:367-81.
111. Wilson M, Barrell G. Modification of a method for cannulation of the cisterna magna in sheep to enable chronic collection of cerebrospinal fluid. Lab Anim 2015;49:85-7.
112. Falconer J, Owens PC, Smith R. Cannulation of the cisterna magna in sheep: a method for chronic studies of cerebrospinal fluid. Aust J Exp Biol Med Sci 1985;63 (Pt 2):157-62.
113. Scott CJ, Tilbrook AJ, Rawson JA, Clarke IJ. Gonadal steroid receptors in the regulation of GnRH secretion in farm animals. Anim Reprod Sci 2000;60-1:313-26.
114. Clarke IJ. Multifarious effects of estrogen on the pituitary gonadotrope with special emphasis on studies in the ovine species. Arch Physiol Biochem 2002;110:62-73.
115. Tilbrook AJ, Clarke IJ. Negative feedback regulation of the secretion and actions of gonadotropin-releasing hormone in males. Biol Reprod 2001;64:735-42.
116. Clarke IJ. The GNRH/gonadotropin axis in the ewe, cow and sow. Domest Anim Endocrinol 1989;6:1-14.
117. Nestor CC, Bedenbaugh MN, Hileman SM, Coolen LM, Lehman MN, Goodman RL. Regulation of GnRH pulsatility in ewes. Reproduction 2018;156:R83-99.
118. McCosh RB, Lopez JA, Szeligo BM, et al. Evidence that nitric oxide is critical for LH surge generation in female sheep. Endocrinology 2020;161:bqaa010.
119. Wickramasuriya N, Hawkins R, Atwood C, Butler T. The roles of GnRH in the human central nervous system. Horm Behav 2022;145:105230.
120. Wang F, Flanagan J, Su N, et al. RNAscope: a novel in situ RNA analysis platform for formalin-fixed, paraffin-embedded tissues. J Mol Diagn 2012;14:22-9.
121. Merkley CM, Renwick AN, Shuping SL, Harlow K, Sommer JR, Nestor CC. Undernutrition reduces kisspeptin and neurokinin B expression in castrated male sheep. Reprod Fertil 2020;1:1-13.
122. Christian P, Smith ER. Adolescent undernutrition: global burden, physiology, and nutritional risks. Ann Nutr Metab 2018;72:316-28.
123. Harlow K, Griesgraber MJ, Seman AD, et al. The impact of undernutrition on KNDy (kisspeptin/neurokinin B/dynorphin) neurons in female lambs. J Neuroendocrinol 2022;34:e13135.
124. Aerts EG, Harlow K, Griesgraber MJ, et al. Kisspeptin, neurokinin B, and dynorphin expression during pubertal development in female sheep. Biology 2021;10:988.
125. Wood S, Loudon A. Clocks for all seasons: unwinding the roles and mechanisms of circadian and interval timers in the hypothalamus and pituitary. J Endocrinol 2014;222:R39-59.
126. Esposito E, Li W, T Mandeville E, et al. Potential circadian effects on translational failure for neuroprotection. Nature 2020;582:395-8.
127. Piccione G, Giannetto C, Casella S, Caola G. Circadian activity rhythm in sheep and goats housed in stable conditions. Folia Biol 2008;56:133-7.
128. Wyse CA, Zhang X, McLaughlin M, et al. Circadian rhythms of melatonin and behaviour in juvenile sheep in field conditions: effects of photoperiod, environment and weaning. Physiol Behav 2018;194:362-70.
129. Plaza J, Palacios C, Abecia JA, Nieto J, Sánchez-garcía M, Sánchez N. GPS monitoring reveals circadian rhythmicity in free-grazing sheep. Appl Anim Behav Sci 2022;251:105643.
130. Helm B, Ben-Shlomo R, Sheriff MJ, et al. Annual rhythms that underlie phenology: biological time-keeping meets environmental change. Proc Biol Sci 2013;280:20130016.
131. Tsingotjidou A, Papadopoulos GC. Neuronal expression of Fos-like protein along the afferent pathway of the milk-ejection reflex in the sheep. Brain Res 1996;741:309-13.
132. Tsingotjidou AS, Papadopoulos GC. Anatomic organization of the ascending branch of the milk-ejection reflex in sheep: primary afferent neurons. J Comp Neurol 2003;460:66-79.
133. Ricordeau G, Martinet J, Denamur R, Petrequin P, Carpentier M. Traite a la machine des brebis préalpes du sud. importance des différentes opérations de la traite. (in French). Ann Zootech 1963;12:203-23.
134. Kosmidis EK, Tsingotjidou AS, Iliadis L, Batzios C, Papadopoulos GC. A sparsely connected network to model the relay stations of the sheep milk ejection reflex. Neurocomputing 2009;73:80-6.
135. O’Callaghan TF, Ross RP, Stanton C, Clarke G. The gut microbiome as a virtual endocrine organ with implications for farm and domestic animal endocrinology. Domest Anim Endocrinol 2016;56 Suppl:S44-55.
136. Kraimi N, Dawkins M, Gebhardt-Henrich SG, et al. Influence of the microbiota-gut-brain axis on behavior and welfare in farm animals: a review. Physiol Behav 2019;210:112658.
137. Forcina G, Pérez-Pardal L, Carvalheira J, Beja-Pereira A. Gut microbiome studies in livestock: achievements, challenges, and perspectives. Animals 2022;12:3375.
138. McDonough CM, Xu HS, Guo TL. Toxicity of bisphenol analogues on the reproductive, nervous, and immune systems, and their relationships to gut microbiome and metabolism: insights from a multi-species comparison. Crit Rev Toxicol 2021;51:283-300.
139. Ammerman CB, Miller SM, Fick KR, Hansard SL 2nd. Contaminating elements in mineral supplements and thier potential toxicity: a review. J Anim Sci 1977;44:485-508.
140. Soliman SA, Svendsgaard D, Farmer JD, Curley A, Durham WF. Six-month daily treatment of sheep with neurotoxic organophosphorus compounds. Toxicol Appl Pharmacol 1983;69:417-31.
141. Evans NP, Bellingham M, Sharpe RM, et al. Reproduction Symposium: does grazing on biosolids-treated pasture pose a pathophysiological risk associated with increased exposure to endocrine disrupting compounds? J Anim Sci 2014;92:3185-98.
142. Guignard D, Canlet C, Tremblay-Franco M, et al. Gestational exposure to bisphenol a induces region-specific changes in brain metabolomic fingerprints in sheep. Environ Int 2022;165:107336.
143. Shen X, Chi Y, Xiong K. The effect of heavy metal contamination on humans and animals in the vicinity of a zinc smelting facility. PLoS One 2019;14:e0207423.
144. Pinnapureddy AR, Stayner C, McEwan J, Baddeley O, Forman J, Eccles MR. Large animal models of rare genetic disorders: sheep as phenotypically relevant models of human genetic disease. Orphanet J Rare Dis 2015;10:107.
145. Kalds P, Zhou S, Cai B, et al. Sheep and goat genome engineering: from random transgenesis to the CRISPR era. Front Genet 2019;10:750.
146. Banstola A, Reynolds JNJ. Mapping sheep to human brain: the need for a sheep brain atlas. Front Vet Sci 2022;9:961413.
147. Murray SJ, Mitchell NL. The translational benefits of sheep as large animal models of human neurological disorders. Front Vet Sci 2022;9:831838.
148. Kendrick KM. Response to 'Sheep recognize familiar and unfamiliar human faces from two-dimensional images'. R Soc Open Sci 2019;6:182187.
149. Gerussi T, Graïc JM, Grandis A, Peruffo A, Cozzi B. The orbitofrontal cortex of the sheep. Topography, organization, neurochemistry, digital tensor imaging and comparison with the chimpanzee and human. Brain Struct Funct 2022;227:1871-91.
150. Murray SJ, Black BL, Reid SJ, et al. Chemical neuroanatomy of the substantia nigra in the ovine brain. J Chem Neuroanat 2019;97:43-56.
151. Guidelines for the housing of sheep in scientific institutions. Available from: https://www.animalethics.org.au/__data/assets/pdf_file/0010/249913/Guide-23-housing-sheep.pdf. [Last accessed on 25 Oct 2023].
152. Cornell University College of Veterinary Medicine. Basic biosecurity best management practices for sheep & goat farms. Available from: https://www.vet.cornell.edu/animal-health-diagnostic-center/programs/nyschap/modules-documents/basic-biosecurity-best-management-practices-sheep-goat-farms. [Last accessed on 25 Oct 2023].
153. Allen MJ, Borkowski GL. The laboratory small ruminant. 1st ed. CRC Press. Available from: https://doi.org/10.1201/9780849377518. [Last accessed on 25 Oct 2023].
154. Cost to keep a sheep for one year in city limits. Available from: http://www.tvsp.org/cost_to_keep_a_sheep.html?fbclid=IwAR3PW02mmzhI1y2_BpejoJoZz4D4dJAhG_tiSC6Iu6X0YPU6FkwA74Q4wqA. [Last accessed on 25 Oct 2023].
Cite This Article
Export citation file: BibTeX | RIS
OAE Style
Chatzimanou T, Tsingotjidou A. A one-health approach to using sheep in research, with a focus on neuroscience studies. One Health Implement Res 2023;3:107-24. http://dx.doi.org/10.20517/ohir.2023.13
AMA Style
Chatzimanou T, Tsingotjidou A. A one-health approach to using sheep in research, with a focus on neuroscience studies. One Health & Implementation Research. 2023; 3(4): 107-24. http://dx.doi.org/10.20517/ohir.2023.13
Chicago/Turabian Style
Chatzimanou, Tryfon, Anastasia Tsingotjidou. 2023. "A one-health approach to using sheep in research, with a focus on neuroscience studies" One Health & Implementation Research. 3, no.4: 107-24. http://dx.doi.org/10.20517/ohir.2023.13
ACS Style
Chatzimanou, T.; Tsingotjidou A. A one-health approach to using sheep in research, with a focus on neuroscience studies. One. Health Implement. Res. 2023, 3, 107-24. http://dx.doi.org/10.20517/ohir.2023.13
About This Article
Special Issue
Copyright
Data & Comments
Data
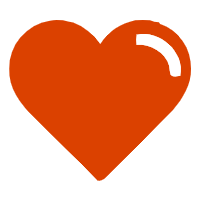

Comments
Comments must be written in English. Spam, offensive content, impersonation, and private information will not be permitted. If any comment is reported and identified as inappropriate content by OAE staff, the comment will be removed without notice. If you have any queries or need any help, please contact us at support@oaepublish.com.